Engineering the Future: Nanoparticles Part 3 - Good Nanoparticles
How can we leverage the nature of nanoparticles for good? What materials science and medical applications exist?
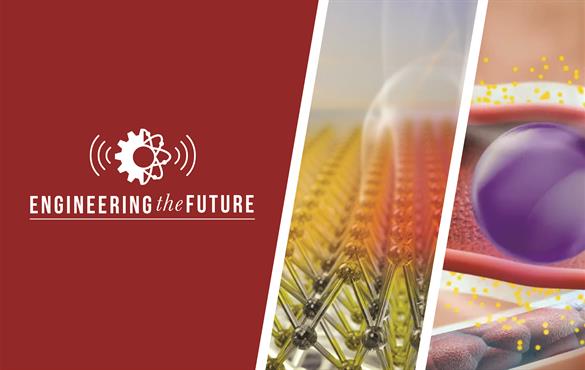
Podcast Host
Aaron Bobick
Dean
Episode 4 Guests
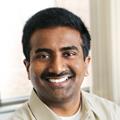
Professor
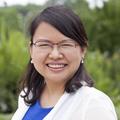
Assistant Professor
This is the fourth episode of Dean Aaron Bobick’s new podcast: Engineering the Future.
How are engineered particles used for good in medicine and materials science?
Transcript
From Washington University in St. Louis's McKelvey School of Engineering, I'm your host, Dean Aaron Bobick, and this is Engineering the Future, where we explore pressing problems of today in which engineering discovery, innovation, and education can provide solutions for tomorrow. Welcome to the third episode of our four-part series on nanoparticles. First we introduced nanoparticles and spoke a bit about what they are and how they're made. In the second episode we discussed some of their more hazardous properties in terms of impact on the environment and human health. And frankly, it was kind of scary. So to close out the series, we have two episodes that are a bit more positive. First we'll be discussing the use of engineered nanoparticles in various domains ranging from medicine to material science. So first we'll begin with some medical applications. Returning to chat with us is Srikanth Singamaneni, professor in the mechanical engineering and material science department who has developed nanoparticles that can be used to enhance a variety of medical diagnostic assessments. Thanks for coming back, Srikanth.
Thank you for having me.
So when we last spoke, you described both how nanoparticles interact with light in interesting ways and also how you can produce these particles essentially by chemistry. But I know you've been quite involved in developing technologies that harness these particles to make certain types of diagnostic tests easier to perform. So what types of tests are we talking about?
So we are primarily interested in improving medical diagnostics that rely on looking at specific biomolecules in physiological fluids. For example, blood, urine, saliva.
So looking for particular proteins or other things that express themselves in these common fluids?
The common word for this is molecular biomarkers, as they call it. Basically, biomolecules that are present in our physiological fluids that are indicator of diseases.
Okay. And so what are the tests that we do?
So one of the common tests that is done for protein biomarkers is called ELISA, enzyme-linked immunosorbent assay, basically trying to determine the concentration of a particular protein in physiological fluid. I can give you a specific example. So when a patient complains of chest pain and when there is a suspicion that he might be going through a heart attack, myocardial/ infarction, so one of the proteins that is present at higher concentrations in the blood is called cardiac troponin, and the cardiac troponin levels-- higher levels of this cardiac troponin that is definitely indicator of a heart attack.
So what you need to do is draw some blood from the patient and then somehow try to figure out what the level of this protein is?
Yes.
Okay. So how is that test done?
So that test is done through a technique called enzyme-linked immunosorbent assay, which involves the use of antibodies to capture the cardiac troponin, and subsequently you go through an enzymatic amplification process to intensify the optical signal, and finally to determine the concentration of the cardiac troponin.
So it reacts to light in some way when you say optical signal?
Right. So in this particular case, that ELISA test I was talking about, you are measuring the intensity of the color of a particular compound.
So that seems like a perfectly reasonable thing to do. What's the problem?
Yeah, unfortunately, the test actually relies on expensive piece of equipment and it needs specially trained personnel to run these tests. So that is a challenge.
So to save the day is going to be nanoparticles. So tell us a little bit about what tests can be done using some of the techniques that you've developed.
So one of the techniques that we are working on right now is called fluorescence amplification using plasmonic nanostructures. Fluorescence is also, again, an optical signal where you are shining one wavelength of light and these molecules, these fluorophores, as they are called, emit a different wavelength of light, and by measuring the intensity of this second wavelength of light that is being emitted, you quantify the number of molecules that are present.
So the biomolecule that you're looking for, itself, will respond to these wavelengths of light? Or do you have to attach something to them to respond?
Yes. It is attached. This fluorophore is-- this emitter is attached to these molecules.
So you treat the sample with these emitters, and then you shine light on it, and then they fluoresce?
Yes. The problem with that is this technique is not very sensitive. It cannot match with that enzymatic amplification that I was talking about previously. That's why fluorescence-based techniques are not used, especially when you want to detect extremely low levels of these proteins or these biomarkers.
And so how does the work in your lab and nanoparticles change that?
We are using plasmonic nanoparticles to enhance the fluorescence that is emitted by these molecules. What we do is take these gold nanoparticles-- of course, shape-controlled gold nanoparticles in close proximity to these fluorophores, and once we take these gold nanoparticles close to these fluorophores, the signal from the fluorophore is amplified by almost 1000 times, and that makes it even more sensitive compared to the enzymatic amplification that I was talking about previously.
So to step through this, so you attach these molecules to the substance you're trying to detect. Those molecules themselves fluoresce under light excitement of a particular frequency, but they only fluoresce a certain amount. To make it more visible, you need to amplify that in a particular way, and because these gold nanoparticles react to this light in a particular way, they get that amplification.
Right. So the nanoparticles are serving as antennas for visible light and the near-infrared light.
Good. That answers a question I was about to ask of-- it's not like these things are plugged in, so you can't actually amplify. You can't put energy into the system. But what you're basically must be doing is concentrating the photons--
Precisely.
--in a way that makes them more visible.
Yes.
Right. And so what type of technology or-- you talked about it being an expensive piece of equipment. Does this require expensive equipment in order to do this new step?
No. This is just a simple patch-like material. It's almost like adhesive tape that we have developed, and all you have to do is just roll this tape on top of these substrates where you have these fluorophores previously, and that immediately amplifies the signal by at least 100 to 1000 times.
So this doesn't sound that complicated or expensive.
Yeah, it is not complicated. And the patch itself, the adhesive I was talking about, is only maybe 10 cents per [inaudible].
So this makes this type of technology available in environments that have, say, many fewer resources to do medical testing?
Yes. Precisely. So previously you had to rely on expensive readout equipment because you need your detectors to be sensitive enough to pick up that light. But because now the light is being amplified by the plasmonic nanoparticles, so you don't need as expensive detectors anymore, and you can possibly use smartphone devices to be able to pick up the signal.
So that's a very interesting use of nanoparticles in diagnostics. I know that they're also being incorporated into actual treatments, and in particular, cancer treatments. Can you say a little bit about what that entails and what that means?
So again, taking the same example of plasmonic nanostructures as gold nanoparticles, the nanoparticles also have the ability to concentrate light and convert part of that light into heat. So you can, in theory, take these particles and deliver them to the tumors, and now when you shine light on these tumors, the particles which are inside the tumors absorb this light, convert that light into heat, and start killing the tumor cells locally without hurting the healthy tissues around.
So the heat directly kills the cancer?
Yes.
Is that commonly used?
It is being explored. Especially this particular technique is under clinical trials for especially head and neck tumors right now.
And are there other ways that we can leverage those particles?
Yes. So you can also use the particles as delivery vehicles, where you load these particles with cargo, that being the therapeutic, the drug.
So these gold nanoparticles, you can make them hollow so they can actually carry a drug with them?
Yes.
All right. And then you got to get the particles to the tumor. So I guess we inject them in the bloodstream. And how do we get the particles to go to the tumor?
So the blood vessels that grow in the tumor-- that are present in the tumor are leaky. They're not perfect, so there are tiny holes in the blood vessels. So the particles tend to leak from those holes and then enter the tumor. That is one way. And you also want these particles to attach to the tumor cells, so you modify the particles with some biomolecules, say, for example, antibodies, which can specifically recognize the proteins that are present on the tumor and then attach to those locations.
Right. So if I understand, basically we take those nanoparticles. Sometimes we leave them alone and we're just going to illuminate them and they'll generate heat to kill the tumor. Sometimes they carry therapeutics with them, in which case the light which will generate the heat will activate the therapeutics. And then we have to get the particles to affix themselves to the tumor, and we can do that by modifying the surface with, say, for example, antibodies that have been designed to target that tumor.
Right.
Well, that's great. Thanks again for joining us. I always love learning what's going on in your lab, and it's really remarkable.
My pleasure.
[music]
My conversation with Srikanth brought up the notion of using nanoparticles to treat tumors. Now one of the most insidious types of tumors are the brain tumors because of, obviously, the challenge in terms of operating on them. And I know that there's this general problem of getting drugs to the brain. There's this so-called blood-brain barrier. So to understand more about that challenge and some possible technological approaches to overcoming, I was fortunate to be able to grab Professor Hong Chen, who is a professor both in the biomedical engineering department here at Wash U and as part of the medical school in the radiation oncology department. Welcome, Hong. Thanks for coming.
Thank you for giving me this opportunity to talk with you.
So Hong, one of our colleagues, Srikanth Singamaneni, spoke with me a little bit about using nanoparticles for treatments and diagnostic tests, and he talked about using them for treating tumors. And we talked about injecting them in the blood and how they have to affix themselves, and he talked about the blood vessels leaking into the tumors and they get there. But I know that when faced with brain tumors that this is a real problem. Can you just describe a little bit about what the actual challenge is in terms of getting drugs into the brain?
The major challenge of drug delivery to the brain, as you already mentioned, is the blood-brain barrier, and this barrier-- you can consider it is a plastic wrap that wrap around all the blood vessels in the brain that normally serve the purpose of protect our brain from any toxin in the blood. But when it goes to treatment of brain diseases, for example, brain cancer, as well as other brain diseases, it become a critical barrier. So the drug couldn't cross it.
So if there are some chemicals or something in your blood that would be particularly bad for brain tissue, this blood-brain barrier protects the brain from being exposed to those particular chemicals?
Yes.
But I guess if the chemicals in the blood are good ones, that is, drugs we've put in there on purpose, then this barrier actually becomes a problem.
Exactly. And that's where we needed novel techniques to overcome this barrier to enhance the delivery of nanoparticles to the brain to make good use of the nanoparticles as a therapeutic tool.
So I will admit that I was a little bit surprised when I first heard about the work you were doing recently in this, because I know you as an ultrasound expert, someone who's working on new modalities of imaging and managing to leverage ultrasound in interesting ways. But I guess this is an ultrasound method that you've been pursuing in order to try to overcome this blood-brain barrier problem. So tell us a little bit about what you and your colleagues have been doing.
Yeah. When we talk about ultrasound, the first reaction that everyone has is ultrasound imaging, but the application of ultrasound in the past 50 years has been broadly expanded into the therapeutic region where we use ultrasound as a therapeutic tool to treat diseases beyond just use it to diagnose disease.
So you actually use the sonic energy that ultrasound emits to cause change within the tissues of the body somehow?
Yes, exactly. So we use the sound energy which, we all know, is safe. There's no radiation involved. It can penetrate deep into our body, and specifically we have way to localize the sound energy to a tiny localized spot. In this way, we can achieve non-invasive localized and targeted therapy. And then in terms of the change ultrasound can generate in our body, currently we are utilizing the mechanical effect of ultrasound as a way to mechanically open the blood-brain barrier.
So we have to get the nanoparticles to this tumor, and you're going to help using ultrasound somehow. So explain to me how you've been using ultrasound to enable the use of nanoparticles for the treatment of brain tumors.
So to answer that question, I'm going to step back one step to talk about this intranasal drug delivery.
Intranasal?
Yes. So deliver drugs directly from the nose to the brain. So the key point here is in our nose there are olfactory nerves and trigeminal nerves. Those nerves directly connect our nose to the brain.
So there's a direct connection between tissue that's used for the sense of smell and the brain?
Yes. Exactly. So we utilize that pathway to deliver nanoparticle from the nose to the brain and bypass the systemic circulation. So because for nanoparticle delivery, one of the main concern is safety.
So by providing it in through the nasal cavity through a spray or drop, it sort of is absorbed directly into the brain tissue without being systemically delivered through the blood system?
Exactly. So we were able to radiolabel those nanoparticles and then use path imaging to track the distribution of the nanoparticle in the whole body of the mouse and then validate that there's not much accumulation of the nanoparticle in liver, kidneys, spleen, and most of them actually we can locally deliver to the brain.
You're Dr. Ultrasound. So where does ultrasound come into this?
Exactly. So intranasal delivery is not new. Is a technique that has been studied for several decades. It was even used in clinical trial for deliver insulin to the brain for treating Alzheimer's disease, but it never go very far. The reason is because delivery efficiency is very low. You can imagine you gave the drug to the nose. Only a very little amount of the drug go to the brain. And second, drug that reach the brain will spread into the whole brain. It won't be localized to where the diseased region is. So that's where ultrasound play the magic.
Okay. So the particles go in through the nasal cavity. They don't have to worry about the blood-brain barrier because they essentially go directly through the nasal pathways. But now you have this problem that they're in the brain generally and you need to get them targeted or focused at a particular region.
Exactly.
And then you're going to use ultrasound to make that happen?
Yes. So the way that we do it is first we use focused ultrasound. So a ultrasound technique that can localize or focus the ultrasound energy to a focal point. It works in a similar way as using a magnifying glass to focus sunlight. So you can focus sunlight to a localized spot.
Kind of like an ultrasound lens.
Exactly. So that's the first element. Second element is the microbubbles. So the microbubbles are bubbles, but smaller in size. They are already used in the clinic as ultrasound contrast agents for ultrasound imaging. But what is a magic that microbubbles can play is when you apply ultrasound to these gas bubbles, they oscillate. So they expand and contract. They generate mechanical force to the blood vessel wall and into the surrounding tissue.
So the contrast agent that we use for ultrasound imaging actually has this microbubble structure, and those respond in an interesting way to ultrasound energy?
Yep.
And normally we use that in order to get different contrasts for imaging, but you're going to use it to have a mechanical effect on the local tissue?
Yes. Exactly.
And you said they expand and contract. And why is this an important thing for this nanoparticle drug delivery?
This is important because our fundamental hypothesis is that the microbubble expansion and the contraction generate this effect we call the microbubble pumping effect. So the microbubble pumping effect leads to enhanced accumulation of the nanoparticle at where the focused ultrasound is applied.
What does the pumping cause to happen in the brain area?
So for drugs that go from nose to the brain, what we find is that the nanoparticles actually travel along the blood vessels, but outside the blood vessel. So consider--
So they flow along the outside of the blood vessels?
Yes. Exactly. And the way that they distribute in the whole brain is mainly through the heart-pumping-generated paravascular pumping effect. So when your heart pump, your blood vessel has pulse wave, and then that pulse wave actually push the nanoparticle to travel along and distribute in the brain. And then based on that fundamental information that we learned, now our observation is the microbubble can also generate this pumping effect. So we hypothesize that the microbubble pumping effect can leads to enhance the delivery and accumulation of nanoparticle.
So basically you've managed to get the nanoparticles into the brain tissue, if you will, but the way those particles move through the brain is essentially along the pathways that contain the blood vessels. They're not in the blood vessels, but they're moving along the blood vessels. The blood vessels themselves actually expand and contract naturally from the heartbeat, but you're going to provide an artificial stimulation which will cause more nanoparticles to move to the area where you're doing that pumping, and you've concentrated the ultrasound action to be in the location where the tumor is.
Exactly.
That is so cool. And so you've done this on-- you've been doing this on mice?
Yes. Exactly. We have proved the concept in mice, and we are also planning to test it in larger animals.
What's the biggest technical challenge to moving to larger animals?
So one of the large challenge-- or the technical challenge is larger animal has thicker skull, so in terms of design of the ultrasound system, we have to consider how to compensate for the attenuation and the scattering of the skull. So the goal is design ultrasound system that can still focus the ultrasound energy to the target location.
So you basically need a better ultrasound lens for thick-skulled people like me [laughter]. Well, listen. That's terrific. That's very cool. It's very exciting. I know that there are many cancer diseases, especially among children, where the brain tumors are inoperable and that being able to treat them like this would be just critically important. So get back in the lab and get to work.
Exactly.
All right [laughter]. Thank you so much for joining us.
Thank you. Thank you.
[music]
That concludes this episode about using nanoparticles in medical applications. Some of the work, like that by Professor Singamaneni, is almost ready to be deployed today. Others, like that of Professor Chen, is a bit further out. But both represent significant advances in medicine made possible by nanoparticles. Before I go, I want to mention that if you were paying attention, this is the first time you've heard the name McKelvey Engineering, as we just had the opportunity to name the school in honor of Wash U Engineering alum Jim McKelvey. Some of you will remember that Jim's dad, also James McKelvey, was dean of engineering for many years, so having the school named after his son of the same name is delightful. Join me next time for our final episode about other engineered uses of nanoparticles. Until then, this is Dean Aaron Bobick of the McKelvey School of Engineering at Wash U. Thanks for listening.