Engineering Interdisciplinary Neuroscience with ShiNung Ching
ShiNung Ching tackles complex problems in brain science with collaborators across disciplines from psychology to systems engineering
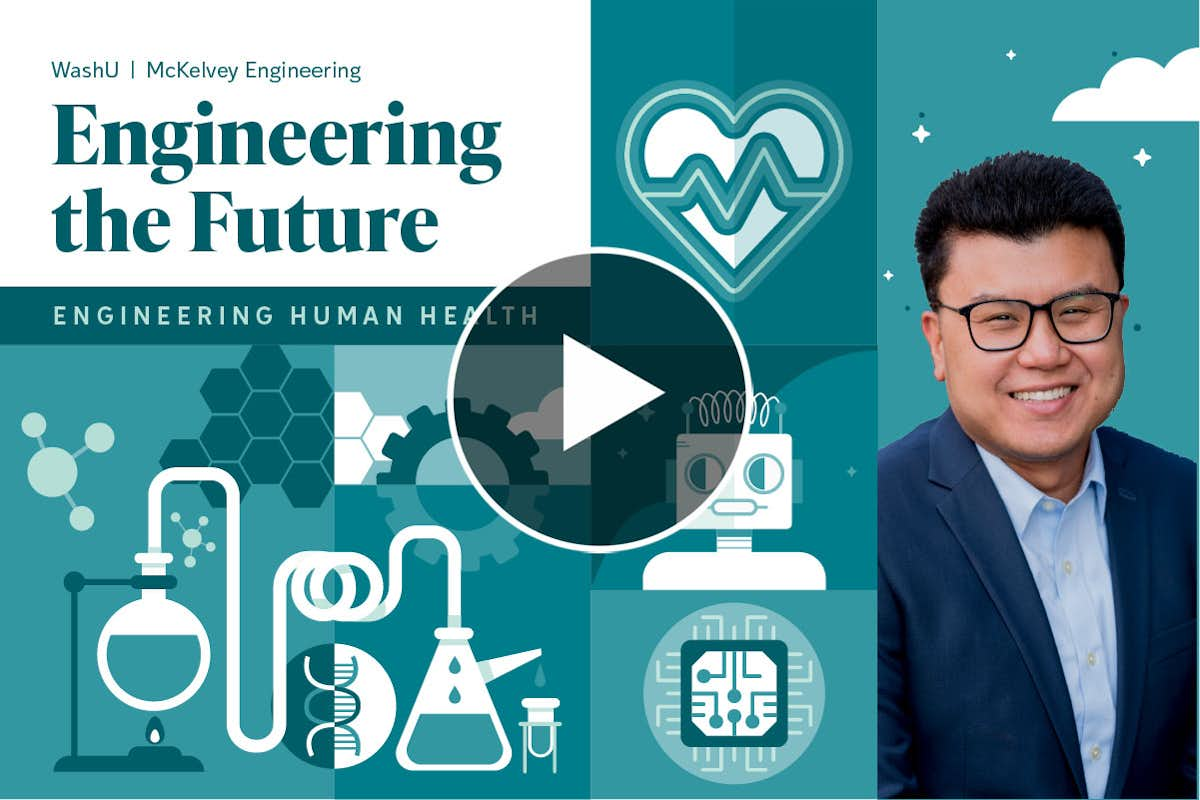
In the latest episode of Engineering the Future, host Shawn Ballard dives into the realm of brain science and the future of mental health with guest ShiNung Ching, associate professor in the Preston M. Green Department of Electrical & Systems Engineering. They explore the intersection of engineering and neuroscience, discussing the complexities of understanding brain functions, the challenges of interdisciplinary research, and the promising future of neuroengineering in addressing mental health issues. Listen in to learn how advanced models and technologies are being developed to enhance cognitive function.
ShiNung Ching: One of the things that's become clear is that any approach that's going to succeed in this area has to succeed in spite of the tremendous variability that exists across people.
Shawn Ballard: Hello, and welcome to Engineering the Future, a show from the McKelvey School of Engineering at WashU. This season we're focused on engineering human health. I'm your host, Shawn Ballard, science writer, engineering enthusiast, and part-time podcast host. Today, I am here with ShiNung Ching, who is an associate professor in the Preston M. Green Department of Electrical & Systems Engineering and the leader of the interdisciplinary brain dynamics and control research group here at WashU. Welcome ShiNung.
SC: Thank you, pleasure to be here.
SB: I am so delighted to get to pick your brain about brain science. I will lean into that pun there. Your work is at the intersection of engineering and brain science. Can you paint me a picture of how those two fields that seem potentially very far apart fit together?
SC: Sure, sure. So, you know, I think one of the features of engineers is that we have an innate kind of interest and desire to understand how things work, right? You know whether that be some robot that you play with as a child or some musical instrument that maybe you're learning. So that curiosity to sort of break it down and figure out how all the parts come together in order to achieve the final outcome of what you're experiencing. And I think that that mindset works very well with a domain like neuroscience.
So, you know neuroscience is centered on the question of how the brain works. It's an incredibly complicated question, one that you can tackle from many perspectives. And I think the mindset of an engineer of trying to sort of decompose problems to sort of figure out how the parts come together fits very well with our goal of understanding the brain.
The brain is an incredibly complex system, and there's a long history to neuroscience. I think one of the things that's become clear over the last several decades is that it's a field that benefits tremendously from introducing multiple perspectives. Right, so there's the biological perspective, but what we're seeing now is also that that there is tremendous benefit to bringing in a more technical perspective, thinking about how we can use mathematics and computation as a complement to traditional biology and traditional science to maybe provide some new theories, new perspectives on how networks in the brain, how cells in the brain, are wired together in order to produce such mesmerizing function, our ability to see and hear and think.
SB: Do you find that those various disciplines, right, they bring sort of different approaches to the brain, do those marry pretty smoothly? Or is there like, there seems like there might be translation difficulties between those very different fields, even as it is very useful to look at something that's so complex.
SC: Yeah, it's interesting. When I first started working in neuroscience, I was trained as an electrical engineer, my degrees are all in electrical engineering. When I made the transition to start thinking about problems in the brain, I was mentored by a mathematical neuroscientist named Nancy Kopell, was one of my mentors at the time, and she made the comment to me that when you start working in neuroscience you're gonna be in meetings and conversations with neuroscientists, and you know nothing is going to make sense. It's going to be like listening to a foreign language, and then after a few weeks maybe one out of every eight words will start to make sense. And then after a few years maybe four out of every eight words are gonna make sense. So you know I'm at a point now where maybe every six out of every eight words make sense.
So I think that that translation is really one of the biggest challenges and opportunities in interdisciplinary research. The different fields come with different sort of mindsets and approaches, but they also come with a different language, and I think the better we get at translating across fields the more we realize that some of that is just genuinely just language, and some of the ideas may in fact be quite synergistic, maybe even overlapping in some cases. And as we as we sort of get better at fluidly kind of communicating, I think we surface those synergies and are then able to tackle bigger and more complex problems as a result, right, because you know as I mentioned, understanding how the brain works, it's a huge problem. It touches on all scales of description from the molecular scale all the way up to the whole brain and then getting into very complex issues of cognition. And so we need all those perspectives if we're gonna have any hope to make progress.
SB: Right, and so as you're sort of bringing all those together, working on these complicated problems, could you sort of preview for me what are some of the big questions in the field now? Right like there’s obviously, how does cognition even happen? Feels like one that I still have. Do the scientists still have that too? We're not sure.
SC: Yeah, yeah, I mean these are all the things that the field is wrestling with right now. What are the mechanisms that give rise to complex behavior? Right, I think it's clear that to make progress towards a question like that we need to think at a systems level about the brain, sort of how all the different parts of the network come together. And as much as these are complex functions, the underlying mechanism is likely also quite complex. And I think just untangling that complexity is what the field is wrestling with right now. So, what are the best tools? What are the best experimental designs? What are the best theories? That will allow us to make headway on this problem.
SB: Okay, so that sounds like it's still perhaps at a very maybe fundamental level of understanding how this works. What are maybe the sort of the next layer of problems? I'm thinking about if we could understand better how the brain works, obviously our theme is health, what does that enable us to do sort of particularly maybe in like the mental health care space? Are you thinking about that yet?
SC: I think there are a number of interesting implications. Just to go off of your earlier question, thinking about cognitive function as maybe the pivot point. So many mental health conditions are associated with changes in cognitive function, deficits in cognitive function, memory, executive function. And I think if we can start to understand the substrate for those functions in the brain, the mechanisms that give rise to those functions, then we'll be better positioned to devise new ways of interacting with the brain in clinical contexts. You know, patients with deficits in these functions, maybe we can find new ways of restoring some of those brain mechanisms that may be altered in those diseases
SB: What sorts of diseases? I’m thinking things like Alzheimer's or …?
SC: Yeah, I mean in the mental health domain I sort of think of things like major depressive disorder, treatment resistant depression. These are conditions that have significant societal burden. I believe the burden of these conditions is measured in the tens of billions of dollars in the United States alone. And it's very interesting, right, so something like treatment resistant depression is defined as a condition where conventional therapies have not succeeded, and that underscores the need for pursuing alternative treatment paths. And so if we can generate new hypotheses of how we might be able to interact with the brain in order to maybe address sort of altered brain mechanisms associated with these conditions, it has the potential to have some major impacts.
Now, there's a lot of work to be done. This is, you know, research, but at least there's a hope there that our basic research and understanding the mechanisms could translate into an area where there are some real needs.
SB: What would that – those kind of interventions that you mentioned – what would that look like?
SC: The path to those treatment options has been quite empirical in nature, right, almost like trial and error. Let's try to sort of do a very wholesale modification of brain electrical activity, and see what happens. I mean, that’s sort of an exaggeration, but it's a very empirical kind of approach in some sense. I think one of the things that we're optimistic about is that as we get better theories for how electrical activity in the brain ultimately gives rise to cognitive function that we'll be able to better use treatments like that so that we don't just go in and shock the brain in a blanket way, but maybe we apply a very small amount of current, you know barely perceptible, in a very sort of focused and precise way that addresses just the right you know signals that then address whatever you know phenotypic dimension that we're going for.
In the research domain, we are trying to develop ways of delivering that type of intervention, you know let's say a transcranial electrical stimulation, but to do it in a way that's very principled, that's based on new understanding of how the brain works, new mathematical tools that we have, new engineering theories that we are developing, so that we aren't just saying, “let me shock the brain and see what happens,” that we are coming in with a hypothesis for how we're going to modify brain activity based on our understanding of the relationship between that activity and let's say memory function, for instance.
SB: Okay, so in your lab you're really developing those like detailed models to be able to know like, okay, here's where or how we can intervene, and this is how the whole system is working together, so modifying one part of the system can then affect the change that we want over the rest of the system.
SC: Exactly, exactly. It's a complex system, and what we're trying to do is to develop mathematical models, computational models that can allow us to understand how the system works, so that we can make a prediction that if I apply you know small intervention to a certain area of the brain, we can now predict what the impact is that of that is going to be throughout the brain. And then to try and associate that with a behavioral outcome that would then arise.
SB: Putting like a lot of faith in these like medical practices to work, and these interventions to work, without sort of being very sure what is actually going on underneath there.
SC: Yeah.
SB: So I am both like intrigued and terrified. [laughs]
SC: I don't want to give the impression that one should not have confidence in medical practice. I mean, I've worked, I've had the great fortune of working with some very talented physician-scientists over the years, and I think that these interventions are incredibly safe. I don't think that is the concern. I think that the question is, can we learn as much as we possibly can about what's exactly happening, and through that we can continue the sort of organic process of improving these things in the future. So that's kind of how I look at it.
SB: Okay, so don't be worried about it, we're just making it better?
SC: Absolutely.
SB: So you've mentioned a number of times these very fruitful collaborations that you've had, and so I'm curious, what does that look like now? You know, you've got your lab here at WashU, you're working on this kind of computational modeling of the brain system. Who are you working with here, and what does that look like for your sort of broader research program?
SC: Yeah, you know, for me, as somebody who's in the McKelvey School of Engineering, who's sort of computationally, mathematically inclined, having collaborators is really an important part of my research. Over my time as a faculty member at Washington University, I've worked with a number of extremely talented faculty across the university, thinking about different problems related to the brain sciences. I've worked with faculty within the School of Engineering, like Professor Barani Raman, thinking about mechanisms of olfaction, how we sort of smell.
And then I've worked with faculty in other departments as well. One of my closest collaborators is Professor Todd Braver. He's in the psychological & brain sciences department, and together we're working on problems of trying to think about how complex cognitive functions like cognitive control and memory are implemented through interactions at the whole brain level, how different parts of the brain are sort of communicating with each other to give rise to these functions. And that's related to sort of some of what I was saying earlier of how we can use, you know, sort of knowledge of these whole brain interactions to maybe come up with new interventions that may be able to modify some of these functions, let's say in a clinical context.
I've also had a number of collaborators in our neuroscience department, working on some interesting problems of how the dynamics in networks of neurons are giving rise to different kinds of computations. My collaborator Thomas Papouin and I have a project that I think is going to be a very interesting one, of thinking about how neurons and other types of cells in the brain interact to maybe augment function in a way that maybe has not been fully appreciated by the community over the last several decades. It's a very vibrant community that we have here in neuroscience at Washington University. It spans, as I said, the basic sciences, the basic biology, but increasingly now is including individuals working in computation and theory, and so it's a very exciting time from that perspective
SB: Is there a particular project that you're working on right now that you're most excited about, that you could sort of dig in and share a little bit more with us?
SC: Yeah sure, I guess I'll just continue on this thread of the whole brain modeling project that I've just mentioned, this collaboration with Professor Todd Braver. I think it's provided new capability to understand measurements that we're making from the brain and translate those measurements into mechanisms, right. So we can sort of go from making a high-dimensional sort of recording of brain activity, maybe using a neuroimaging modality or using an electrophysiology modality, and take those recordings and produce a mathematical model, right so something I can simulate on a computer, and use that model to then deduce something about how those signals were generated through the interaction of brain areas. And by doing that, sort of gain an insight into how the functions are being produced within the brain.
And what we're doing now that I think is so exciting is we're now moving towards not just elucidating those mechanisms, but actually trying to modify those mechanisms using things like stimulation, right these ideas that I've just described. So can we come in with a causal manipulation, applying a small amount of current, maybe even applying or coming in with a pharmacological manipulation, and modifying the dynamics in these networks, so that we may be able to improve, you know, let's say a person's memory.
SB: What is sort of the outlook on that? You know sort of as this goes forward, I guess, what sort of, in five to ten years, would you anticipate that having some sort of like clinical application? Or like how far away are we from seeing that in practice?
SC: Yeah, I mean I think the work that I do, you know we do fundamental research, right, but we always have an eye towards eventual sort of translation, particularly for my projects that are sort of maybe adjacent to these kinds of problems, but also some projects that are a little bit more focused and are in fact clinical in nature.
So I have projects that are in the domain of thinking about understanding some of these mechanisms in the context of brain injury, for instance, with colleagues in the Department of Neurology like Professor Terry Kummer, Professor Rej Guerriero, where we're actually looking at patient populations and understanding how some of these mechanisms may be altered in some of these patients, and how we might be able to have some more immediate impacts associated with maybe providing information to clinicians so that they can make better decisions about how to treat these patients.
And so those things have maybe a little shorter runway. The work that I've described about whole brain modeling, I would view the runway of that as being a little bit longer, more sort of doing more fundamental work, just trying to understand the mechanisms, but nonetheless it's something we're always thinking about, right, sort of how can we get to those to those endpoints.
SB: These new devices, treatments, things that are in development. Are there any takeaways from your research so far that you could sort of share with us even as these things aren't sort of quite in practice?
SC: Sure, yeah, I mean we are thinking about new ways of engineering devices to deliver things like electrical current to the brain, but to do it in as minimally invasive a way as possible. And what I mean by that is sort of, there's minimally invasive in the sense that maybe the device is sort of external to the head, but you still want a device that is relatively small, that is barely perceptible, maybe the amount of current that's applied is extremely small, so that a person has no concerns about safety and so forth. And so while we're developing this theory and these models, we are at the same time thinking about how we can eventually pair that with some technology. So we have what I would characterize as early-stage work to think about what kinds of device designs would be needed to actually both test some of these ideas and also bring them into practice.
So in collaboration, you know with my collaborators, we are doing some human studies work to test early versions of this technology, and what I could envision in the future is having devices that one can buy that would deliver a very small amount of electrical current tailored and personalized to the level of an individual that would then help to enhance some dimension of their cognitive function. I think that that would be pretty transformative if we could do it in clinical applications that we’ve spoken about in particular. For individuals who have deficits in their ability to maybe remember things, right, if we could improve that kind of function, I think it could make a big impact.
SB: Okay, would that be like some sort of, I’m imagining like some sort of cap, or …?
SC: I mean it wouldn't even have to be a cap really. I mean it could be like an athletic headband, right, something like this where you would have just a few electrodes where you could apply a small amount of current. You could move around with it. I think this is the kind of thing that would have an impact. It wouldn't require you to be in a clinical setting. You could do it at home, but it would nonetheless, you know, we would have the insight and the computational capacity to tailor that to the individual without having a lot of supervision so to speak.
SB: Okay, so that would be sort of a matter of, you know you've got your model of the brain, the system that you've developed, and then sort of looking at a scan of the individual to tailor? Or how would that work with the tailoring?
SC: For instance, for instance, yeah. So we would do something like you'd have a device that would sort of measure a certain amount of brain activity from the individual and, based on that, produce a characterization of the optimal amount of current and location on the scalp to deliver that current to get the desired effect. So again, I mean the runway on this is long, but I think we are actively thinking about it because in the end, right, the best demonstration of our theories and methods is if we can interact with the system.
SB: Right.
SC: So, you know, the only way to prove correctness in some sense is if we generate a way of interacting with the brain that then we test, and it works. So we are working on this, and it's something we're very excited about. And so that's accompanying the development of the theory.
I think our hope is that through the research that we're doing, to the extent that there's a consumer application for something like this, that we can again you know just come at this from a very principled way that it isn't “try this and it might work,” right. That it's born out of a scientific knowledge and hypotheses.
SB: What should sort of me, other regular consumers, who are interested in this type of technology, what should we be looking for from the neuroscience and neuroengineering community to know that like, “oh, this is happening, it's arrived, now we can enhance our memory, we can enhance our concentration”? How will we know from sort of your perspective that these are legit now?
SC: Yeah, yeah. I mean I think I would say a couple things. One of the challenges, you know, this type of research has been going on for some time. I mean, people have been thinking about are there ways that we can enhance memory and so on. One of the things that's become clear is that any approach that's going to succeed in this area has to succeed in spite of the tremendous variability that exists across people.
So, as we do more work, it's increasingly clear that the person-to-person variation in brain mechanisms is actually quite substantial, so a device that acts in a certain way for everybody will not, I would argue, it’s unlikely to succeed in any kind of robust fashion. And so I think one of the things that we're hoping to see over the next, let's say five to ten years, is some progress on coming up with strategies that generalize in a way across all the population, so that if I show memory enhancement in a cohort of 20 people that I can bring in some random group of people, and it will still work.
So I think those sort of reproducibility studies, large cohorts where we see effects over very large numbers of people, I think that kind of progress will then lead to visible translation. You'll start to see devices start to come out that that that we can buy. And so that's the kind of thing I would look for.
I'll mention one other thing that's a little bit adjacent to this issue of devices, but is nonetheless related to improved understanding of cognitive function just sort of broadly speaking that also intersects engineering. As we get a better understanding of how the brain works and how the brain serves cognitive function, I think we're going to be able to use that in other types of engineered systems, right. So I think about systems and autonomous systems, things like self-driving cars for instance, that come up against real challenges in handling all of the idiosyncrasy in how people behave. Right, you come into an intersection, it’s very hard to predict. I mean, you can predict to a certain degree, but once you get into the edges, it's sort of hard to predict what people are going to do. As we get a better understanding of sort of cognitive function, human behavior, I think we're going to be able to integrate that knowledge into these kinds of engineered systems, and we will start to see progress there as well.
So I think these are the kinds of things that I look forward to.
SB: Yeah, that's fascinating. I hadn't even thought, you know, you have collaborations obviously with folks in psychological & brain sciences and at the med school, but this idea of systems that are just complex systems on their own, even if they're not biological, that this would translate over. Do you feel like that translation will be relatively smooth? Are sort of all complex systems, the features of those are translatable in a way, even if the systems are quite different?
SC: Some of those things are translatable for sure.
SB: Okay.
SC: Yeah, some of the basic mathematical principles, some of the engineering principles are quite generic. It's actually one of the strengths, I think, of electrical & systems engineering is that the mathematical tools that we use, the design principles that we use are not specific to any one domain, right. So the work that I do in computational neuroscience, the work that my colleagues do in imaging, in robotics, a lot of the math is actually the same, and the way that we think about the problems tends to be quite similar as well, so I think one of the things that's beautiful about engineering is the versatility of our methods.
SB: Okay, I love that. I will look forward to the brain sciences meets autonomous robots collab.
SC: Oh yeah, it's a real thing, yeah.
SB: Okay, all right, so thinking about that sort of bright and exciting future, I want to shift to a question I ask every guest on this podcast because I am so hungry for media recommendations. I'm curious to hear from you, what are your favorite media representations in books, TV, movies, wherever, of brain sciences, and why? This is your area of expertise, so I bet you notice them when they're bad. I'll take a good one or a bad one, but if they're like really good ones, I'd be very curious about that.
SC: Sure, sure, yeah. That's an interesting question. You know, I think I certainly do notice when they're bad, and that tends to be many. Yeah, when I was a grad student, sort of at the time we're thinking about some of these problems, that was around the time of the movie Inception.
SB: Oh yeah!
SC: Yeah, which is about implanting memories, right, and I think, just sort of this, at a high level, this fascinating premise. Could we interact with sort of a core brain function like this? Could we ever get to a point where we understand the representation of memories well enough that we could actually manipulate that? Sort of a fascinating question, and at some level is the kind of thing that we are now thinking about, is sort of, what are the representations in the brain that underlie these kinds of kinds of functions?
And when I was first starting my postdoc, we would work on some problems, and you know we sort of sit around and kind of brainstorm, and we were brainstorming about some problems related to brain stimulation in a medical context. And I remember we were laughing because we had sort of written this idea of being able to stimulate the brain at these different locations, and we were laughing and saying, it's science fiction, you know. But this is sort of now, fast forward, and these are the problems we're working on. So, I think it should be the case that things that seem like science fiction end up not being the case, right, that we kind of imagine things and start to pursue them. So, yeah, I don't know. I guess that's not an answer as to it's not a recommendation per se, but just to say that I think it's okay to enjoy these things because in the end they sort of inspire us to maybe think about the questions that we want to then address.
SB: Okay, so even if the movies are terrible, right, there could be a kernel there.
SC: Sure.
SB: Okay. Not that I'm saying Inception is terrible.
SC: No, no. [laughs]
SB: Amazing. Okay, I love that, and I'm excited to see what science fiction you turn into reality when it comes to helping me with my forgetfulness.
SC: Sounds great, sounds great.
SB: Well, thank you so much for coming on the podcast. This was illuminating, and I look forward to hearing what's next.
SC: Pleasure to be here, and thank you for the opportunity.
[Music]