Flu’s clues: A new approach to studying influenza
Clever repurposing of biological tool gives researchers new clues as to how the flu remains so successful
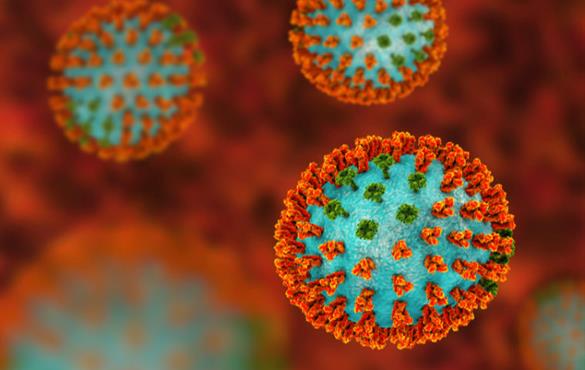
Scientists have known for decades that a flu virus in a human body can be a lot different than viruses grown in a lab. As opposed to the uniform, spherical, textbook-style viruses in a petri dish, in humans they vary in shape and composition — particularly the abundance of certain proteins — even if they are genetically very similar.
It has been difficult however, to study the exact number and location of these proteins on any individual virus. The go-to method in cell biology involves attaching a fluorescent protein to the area of interest; the light makes the area easier to image and study.
But trying to attach fluorescent proteins to the molecules that make up a flu virus is like trying to get a third person on a tandem bike: There just isn’t room. The fluorescent proteins are about the same size as the flu proteins; introducing such a relatively large element throws the virus out of whack.
A paper by Michael Vahey, assistant professor in the School of Engineering & Applied Science at Washington University in St. Louis, and Daniel A. Fletcher, the Purnendu Chatterjee Chair in Engineering Biological Systems and chair of bioengineering at the University of California, Berkeley, demonstrates that flu proteins can be tagged using a different method.
The process has already yielded information that hints to one advantage at minimum for having so many flu phenotypes, that is, various shapes and configurations found in genetically identical flu particles.
The paper was published Nov. 9 in the journal Cell.
“Under what circumstances is it adaptive, and how so?” Vahey asked. “This is a first step toward understanding that. But it’s not a complete picture.”
In order to move past the labeling difficulties, Vahey adapted a method that is typically used to label a specific area on a protein called, appropriately, “site-specific labeling.” Instead of using a fluorescent protein, he inserted sequences five- to 10-amino-acids-long into the proteins that make up influenza A virus. This is the most common flu virus, and also the most dangerous to humans.
After inserting these short sequences, he introduced enzymes and small amounts of fluorescent dyes. These enzymes take different dye molecules and connect them to the engineered viral proteins, giving researchers the ability to see individual proteins without disrupting how they — or the virus they make up — functions.
Of particular interest to researchers are the proteins hemagglutinin (HA) and neuraminidase (NA). HA is responsible for allowing a flu virus to attach to a cell and NA is responsible for decoupling the virus from the cell so that it can go on to infect others. (This is where designations such as H1N1 or H3N2 come from; the surface of the virus has different types of HA and NA that are referred to by specific numbers, or subtypes).
“Once we have the ability to label individual viruses, we can image them and quantify how much of each protein they have per particle, and what the size of that particle is,” Vahey said.
Utilizing site-specific labeling overcomes a longstanding challenge in the study of flu viruses. Now that they could take a more detailed look, Vahey and Fletcher decided to do just that, setting up an experiment that might help them understand whether or not the variation seen in individual flu viruses might be adaptive, helping the virus to spread infection.
The researchers studied individual flu viruses released from cells, some of which were treated with a substance that blocks NA from doing its job, releasing the virus from a cell. (This is how the antiviral drug Tamiflu works. If the virus cannot release itself from the cell, it cannot spread and reproduce).
Then they compared the virus particles that were able to detach from the untreated cells to those that were able to detach from the cells treated with the NA inhibitor.
“What we found is that viruses that are smaller, or have more NA, are more resistant to the NA inhibitor,” Vahey said. “They were more likely to be able to detach from a cell that has been challenged with Tamiflu.” They could then go on to infect more cells.
The results suggest that these two variations — being smaller than average, or having more NA — could be beneficial for a virus that found itself in a person who had been treated with Tamiflu. It’s one example of how having lots of diversity among individual viruses might be advantageous.
On the other hand, viruses with more HA, or that are larger, can bind more strongly to cells. “Under any particular circumstance, it might be beneficial to be anywhere within that range,” Vahey said. “In the case of Tamiflu treatment, you’re inhibiting NA such that the viruses that happen to have more NA and also happen to be smaller now have a little bit of a leg up.”
More broadly, Vahey said, “If you have an environment that is changing rapidly over time, if you were reliant on genetic adaptations, you might be in some trouble, because it takes a certain amount of time for mutations to accumulate.” But phenotypic diversity generates changes relatively quickly. Each time a virus replicates, the next generation displays a host of variation, some of which might be suited to the environment where it finds itself.
Down the line, the importance of phenotype may have implications for the development of new flu vaccines. “Typically in the development of a flu vaccine, you’re concerned about how genetic changes in the virus may reduce the effectiveness of the vaccine,” Vahey said. “This could be an additional consideration, how variation in viral phenotype may contribute.”