Synthetic biology enables protein origami
Fuzhong Zhang, associate professor of energy, environmental & chemical engineering in the McKelvey School of Engineering, and members of his lab have developed a bottom-up approach to build 2D nanostructures, essentially starting from scratch
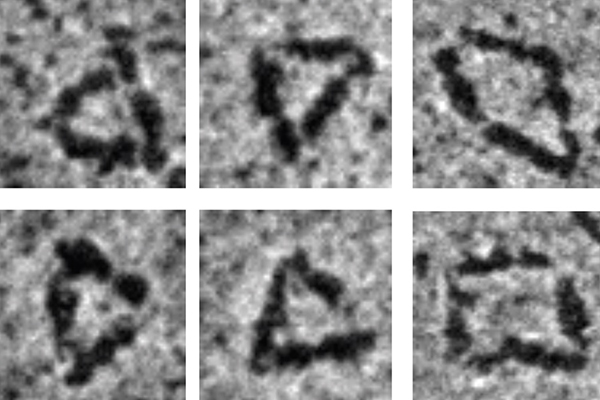
The ancient art of paper folding known as origami is used to make intricate birds or other shapes. Inspired by the work of DNA origami, in which nanostructures are made from folding DNA, a team of engineers at Washington University in St. Louis has found a new way to create single-chain protein nanostructures by using synthetic biology and protein-assembly techniques.
he team created nanostructures — in the shapes of triangles and squares — using stable protein building blocks. These protein nanostructures can endure high temperatures and harsh chemical conditions, both of which are not possible with DNA-based nanostructures. In the future, these protein nanostructures could be used to improve sensing capabilities, speeding chemical reactions, in drug delivery and other applications.
When trying to create protein nanostructures suited for particular applications, researchers typically make modifications to existing protein structures, such as virus particles. However, the shapes of nanostructures that can be made using this approach are limited to what nature provides. Now, Fuzhong Zhang, associate professor of energy, environmental & chemical engineering in the McKelvey School of Engineering, and members of his lab have developed a bottom-up approach to build 2D nanostructures, essentially starting from scratch.
"Building something that nature has not offered is more exciting," Zhang said. "We took individually folded proteins and used them as building blocks, then assembled them together piece by piece so that we can create tailored nanostructures."
The results of the work are published in Nature Communications, July 25, 2019.
Using synthetic biology approaches, Zhang's team first biosynthesized rod-shaped protein building blocks, similar in shape to a pencil but only 12 nanometers long. Then, they connected these building blocks together through reactive protein domains that were genetically fused to the ends of each of the rods, forming triangles with three rods and squares with four rods. These reactive protein domains are known as split inteins, which are not new to Zhang's lab — they are the same tools that his group uses to make high-strength synthetic spider silk and synthetic replicas of the adhesive mussel foot proteins.
In both cases, these split intein groups enable the production of large proteins that make the synthetic spider silk tougher and stronger and the mussel foot proteins stickier. In this case, they enable the construction of novel nanostructures.
Zhang's team worked with Rohit Pappu, the Edwin H. Murty Professor of Engineering, professor of biomedical engineering and an expert in the biophysics of intrinsically disordered proteins, phase transitions and protein folding. Both Zhang and Pappu are members of the university's Center for Science & Engineering of Living Systems (CSELS).
"Professor Pappu's lab, specifically former postdoctoral fellow Jeong-Mo Choi, helped us understand how the protein sequence at the connections determines the flexibility of these nanostructures and helped us to predict protein sequences to better control the flexibility and geometry of nanostructures," Zhang said. "The collaboration between my synthetic biology lab and Professor Pappu's biophysical modeling lab has proven very productive."
The collaboration simplified a very complex process.
"Once we understood the design strategy, the work is fairly straightforward and quite fun to do," Zhang said. "We just controlled the different functional groups, then they controlled the shapes."
Due to the versatile functionality of proteins, these nanostructures potentially could be used as scaffolds to assemble various nanomaterials. To test this idea, the team assembled 1-nanometer gold nanoparticles precisely at the vertices of the triangle. Using a state-of-the-art electron microscope in the university's Institute of Materials Science & Engineering, both the protein triangles and the gold nanoparticles assembled to the vertices of the triangles were visible.
To test the stability of these protein nanostructures, the team exposed them to high temperatures, up to 98 Celsius, to chemicals such as guanidium hydrochloride, and to organic solvents such as acetone. While these conditions generally destroy protein structures, the structures from Zhang's lab stayed intact. This ultra-stability could enable more nanoscale applications that are difficult or not possible using nanostructures made from DNA or other proteins, Zhang said.
Next, the team is working with Srikanth Singamaneni, professor of mechanical engineering & materials science and a member of CSELS, to use these protein nanostructures to develop improved plasmonic sensors.
"Exploiting the interplay between highly stable structural building blocks and intrinsically disordered or flexible regions provides a novel route to designing nanostructures with customizable features for a variety of applications in synthetic biology and biomedical sciences," Pappu said. "This is one of the major thrusts of our center as reflected by the synergies among three different labs that are part of the center."
Bai W, Sargent CJ, Choi J-M, Pappu, RV, Zhang F. "Covalently-assembled, single-chain protein nanostructures with ultra-high stability." Nature Communications, July 25, 2019. DOI: 10.1038/s41467-019-11285-8.
This research was supported by funding from the Office of Naval Research (N000141512515), NASA Space Technology Research Fellowship (80NSSC18K1145), Human Frontier Science Program (RGP0034/2017) and the National Science Foundation (MCB-1614766).