Wobbly molecules get a closer look
Matthew Lew introduced a detailed theoretical framework that allows scientists to describe and measure how biomolecules move more accurately
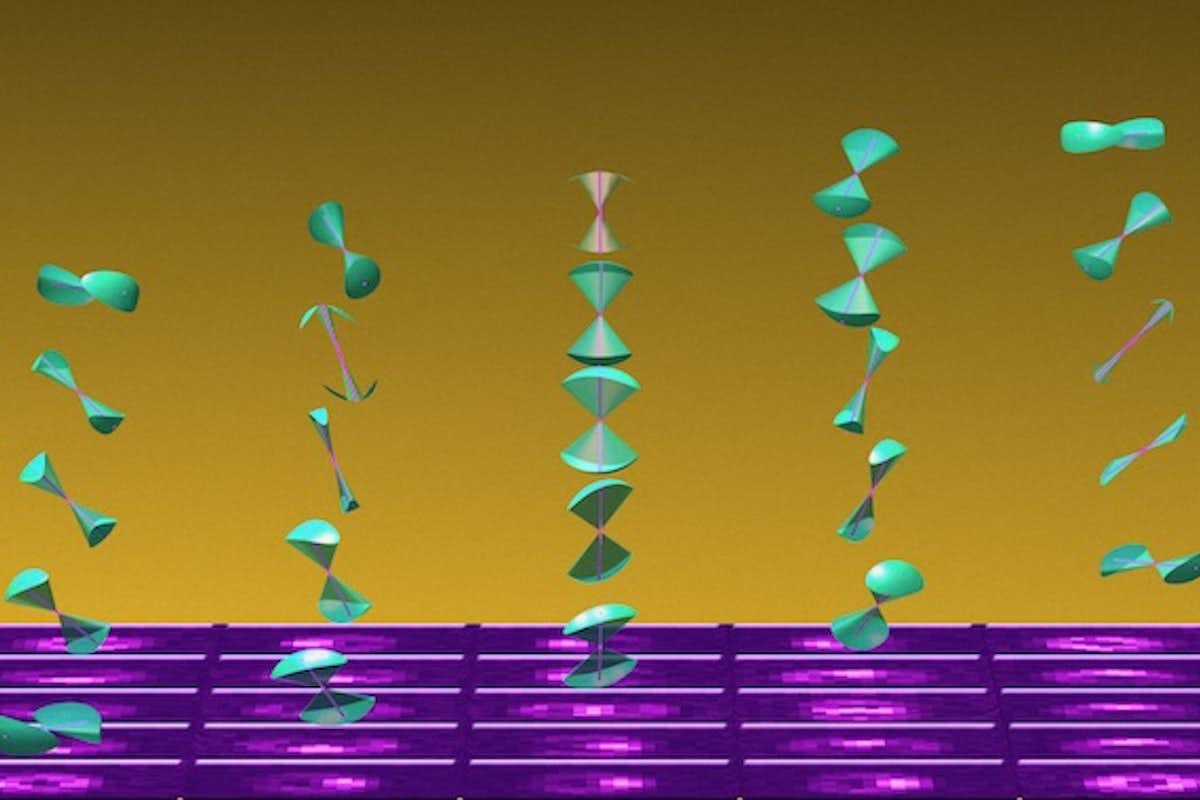
While new technologies, including those powered by artificial intelligence, provide innovative solutions to a steadily growing range of problems, these tools are only as good as they data they’re trained on. In the world of molecular biology, getting high-quality data from tiny biological systems while they’re in motion – a critical step for building next-gen tools – is something like trying to take a clear picture of a spinning propeller. Just as you need precise equipment and conditions to photograph the propeller clearly, researchers need advanced techniques and careful calculations to measure the movement of molecules accurately.
Matthew Lew, associate professor in the Preston M. Green Department of Electrical & Systems Engineering in the McKelvey School of Engineering at Washington University in St. Louis, builds new imaging technologies to unravel the intricate workings of life at the nanoscale. Though they’re incredibly tiny – 1,000 to 100,000 times smaller than a human hair – nanoscale biomolecules like proteins and DNA strands are fundamental to virtually all biological processes.
Scientists rely on ever-advancing microscopy methods to gain insights into these systems work. Traditionally, these methods have relied on simplifying assumptions that overlook some complexities of molecular behavior, which can be wobbly and asymmetric. A new theoretical framework developed by Lew, however, is set to shake up how scientists measure and interpret wobbly molecular motion.
In a cover article published July 18 in the Journal of Physical Chemistry A, Weiyan Zhou, a PhD student in electrical engineering, and Lew introduced a detailed model that allows scientists to describe and measure how molecules move more accurately. Where traditional measurement techniques assume that molecules wobble uniformly in all directions within a circular cone — an isotropic diffusion model – Lew and Zhou discarded this simplification to reflect the true nature of molecular behavior in more complex biological environments.
To get the best data, Lew says, researchers must take into account that real molecules often exhibit anisotropic diffusion, meaning they rotate and translate differently depending on the direction they’re pointing, which is in turn due to their shape or local environment.
“The newest questions and biggest challenges in biophysics right now involve how biomolecules rotate or change their conformations in whatever environment they happen to be in,” Lew said. “We could be talking about a protein after it gets translated from its genetic code, a receptor on a cell surface interacting with a signaling molecule from a neighboring cell or an antibody fighting an attacking virus.
“Optical microscopy has really flourished, to the point where you can watch living things and see how they move and evolve over time, and now the question is, can we add some more detail to see how those biological systems are actually working? How precisely can we measure a molecule’s orientation in realistic environments over time?” Lew said.
Lew’s innovative approach isn’t just about picking up rotational signals more accurately. He and his team also considered how to handle noise and perturbations as well as the quintessentially engineering problem of how instrument design impacts measurement. In practical measurements, random fluctuations caused by quantum shot noise in the image can skew results. Lew’s framework accounts for these systemic inaccuracies and estimates how errors might affect measurements of molecular wobble, paving the way for more precise studies of molecular rotational dynamics in complex biological systems where traditional microscopy simply can’t see the directional details.
This increased precision will be especially valuable in applications like immunology where developing a detailed picture of how antibodies function is critical to understanding their effectiveness, or the study of biomolecular condensates that move, interact, self-organize and evolve over time in ways that scientists still don’t fully grasp. Measuring these biomolecules in motion, not frozen on a microscope slide, is essential, Lew says, to seeing what’s really going on.
“We now know that individual biomolecules have an outsize impact on what’s happening in biophysical systems,” Lew said. “With our new mathematical framework, my lab will be able to design new instruments and new image processing algorithms that account for noise to produce precise, high-dimensional measurements of single molecules. These fundamental developments will have far-reaching effects in helping us paint richer pictures of how biophysical systems work.”
Zhou W, Wu T, Lew MD. Fundamental limits in measuring the anisotropic rotational diffusion of single molecules. The Journal of Physical Chemistry A. July 18, 2024. DOI: https://doi.org/10.1021/acs.jpca.4c03160
This research was supported by the National Institute of General Medical Sciences of the National Institutes of Health (R35GM124858).