Driving force
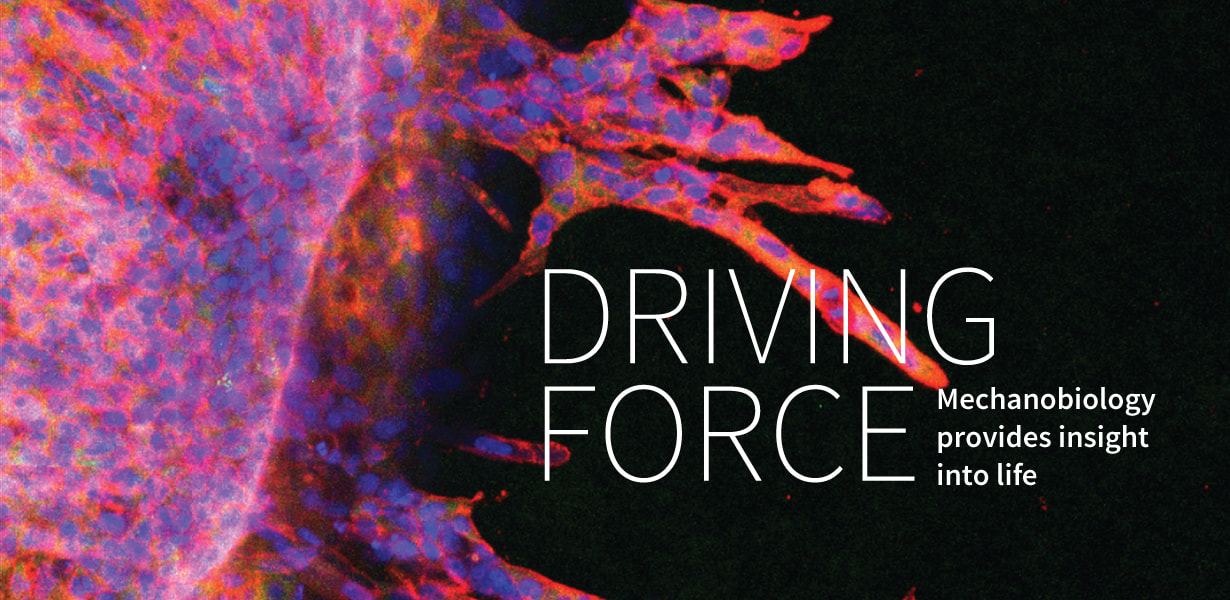
Mechanobiology provides insight into life
In the past few decades in science, major advances have been made in biochemistry, molecular and cell biology, genetics and genomics. However, engineers and scientists have recently identified a missing link that is critical to understanding and improving human health and the living environment — the emerging field of mechanobiology, or how biological systems sense, generate and respond to physical forces. These mechanical forces influence the nervous, musculoskeletal, circulatory and immune systems and play a role in diseases such as hypertension, osteoarthritis and cancer.
The School of Engineering & Applied Science at Washington University in St. Louis, in partnership with the School of Medicine and the School of Arts & Sciences, has long been a leader in mechanobiology, a field that centers around the biological response to mechanical loads on cells and tissue, such as form, function, composition and biochemical and genetic signaling. It covers all living things, which opens opportunities for discoveries in medicine and health, agriculture, systems science and biotechnology.
“Until recently, the field was considered a side concept, and it’s becoming very clear that if you don’t consider the mechanics and look at the biology alone, you may get answers that aren’t exactly right,” said Farshid Guilak, professor of orthopaedic surgery and co-director of the Center of Regenerative Medicine at the School of Medicine, as well as the director of research at Shriner’s Hospitals for Children-St. Louis.
“It’s also opened up whole new areas of research because we’re now understanding that mechanics are necessary for health in certain areas and are actually responsible for disease or degenerative changes in other areas,” Guilak said.
Mechanobiology is a high priority in the school as part of its five-year Strategic Plan, which includes taking advantage of the existing strong group of faculty members in this area, as well as the strong collaborations with faculty members at the School of Medicine and the School of Arts & Sciences, facilities, equipment and students. Adding up to eight new faculty members in mechanobiology, as well as investing resources in doctoral students, postdoctoral fellows and shared equipment will help to raise the school’s influence and visibility in the field both nationally and worldwide, said Jessica Wagenseil, associate professor of mechanical engineering & materials science and co-chair of the school’s Strategic Planning Committee. While the School of Engineering & Applied Science has numerous faculty involved in mechanobiology research, we’ve chosen a few to highlight here.
Development
Mechanobiology plays a role in the earliest stages of development. Organs, including the heart, brain and eyes, require forces to grow and develop into their final shapes. For example, in a chicken embryo, the heart starts as a tube. In half of a day, mechanical forces cause the tube to bend into a C-shape, a process called C-looping, and it begins to pump blood. Over the next 24 hours, forces transform the C-shaped tube into a complete loop with two ends, similar to an awareness ribbon one would pin on a lapel. In humans, the heart begins to beat at about three weeks of gestation, then looping takes about a week, said Larry Taber, the Dennis & Barbara Kessler Professor of Biomedical Engineering and a longtime leader in morphogenesis, or the development and growth aspect of mechanobiology.
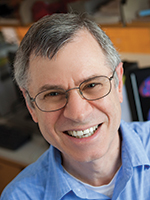
Larry Taber
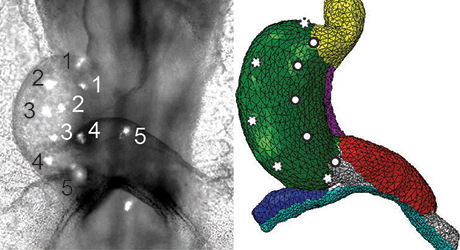
“If organs such as the heart and blood vessels do not experience forces such as blood pressure as they develop, they will never survive normal physiological loads after birth,” Taber said.
While mechanical forces have a necessary influence on development, they also play a role in correcting defects.
“Early on, you’ll see significant differences in heart shape from one embryo to the next,” Taber said. “Somehow it then adapts and acquires the right shape before birth. There seems to be a kind of functional adaptation built in to the embryo that helps it develop in the right way.”
Mechanobiology in disease and injury
While blood pressure can rise when a person is under stress, additional mechanical forces also play a big role: If the pressure increases on an artery, it will grow thicker to help decrease the stress on the artery wall, which will then increase the arterial stiffness and cause a further rise in blood pressure.
Wagenseil’s research focuses on the functions of the large arteries. While Taber studies the development of organs from a tube, Wagenseil studies the maturation and remodeling of the tubes. She and her team manipulate the tubes through genetic modification of extracellular matrix proteins, which alter the mechanical properties of the tube and the resulting form and function.
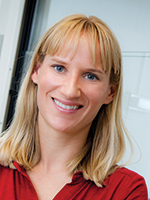
Jessica Wagenseil
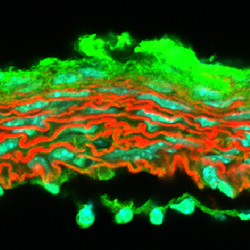
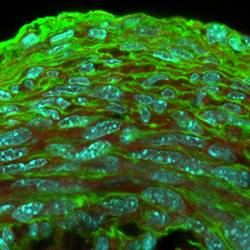
“When the pressure gets higher, the arterial wall remodels,” Wagenseil said. “We’re interested in how that process works, how we can interfere with that process and what the feedback signals are. A lot of the feedback signals depend on the mechanics of the wall — whether it’s stiff or not — and the mechanics are dictated by the amount and arrangement of matrix proteins.”
Wagenseil’s recent work in arterial mechanobiology could change the scope of how heart disease, the leading cause of death in the world, is detected and treated. She showed that increased artery stiffness is a consequence of plaque buildup, not the cause as previously believed. With this knowledge, researchers can take a new look at the relationship among increased plaque and diet, exercise and other lifestyle choices commonly associated with artery health.
Some systems in the body are not mechanical in their normal function, but are influenced by mechanical factors. One of those systems is cancer. Cancer tissues can be up to 10 times stiffer than healthy tissues, which contributes to their survival.
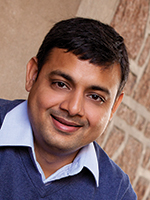
In his research, Amit Pathak, assistant professor of mechanical engineering & materials science, has found that cancer cells can migrate alone or in groups to metastasize. His lab is making different kinds of biomaterials that mimic different properties present in humans, such as stiffness in the environment or geometry, then studying how cells behave in those environments.
In addition to studying cancer, Pathak, who combines material fabrication with cell biology and computational modeling, also is collaborating with faculty at the School of Medicine to devise mechanically optimized platforms for immunotherapy and to study the roles of mechanics in kidney inflammation.
“We are going from mechanics to biology within the same system,” he said. “The input is mechanical by changing the stiffness, while the output is biological, meaning the cells are breaking up. Then you go inside of the cell, find the molecule that’s responsible for that behavior, which may provide new therapeutic targets. That’s the ideal sequence of events in connecting mechanics to biology and therapy.”
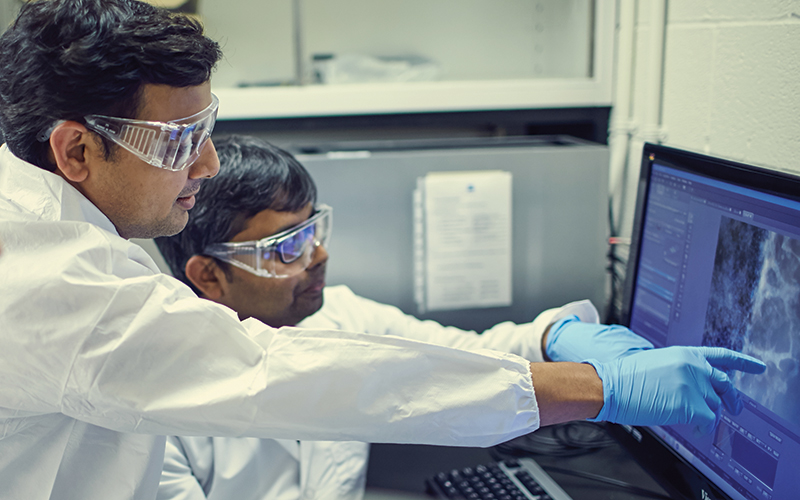
Lori Setton, the Lucy & Stanley Lopata Distinguished Professor of Biomedical Engineering, and Spencer Lake, assistant professor of mechanical engineering & materials science, work closely with the Musculoskeletal Research Center at the School of Medicine. Setton is a renowned researcher in the role of mechanical factors in the degeneration and repair of soft tissues of the musculoskeletal system, including the intervertebral discs, or the cushions between vertebrae that act as shock absorbers, which are an excellent example of mechanobiology in action, Setton says.
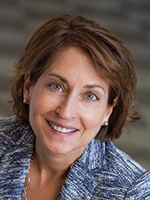
Lori Setton
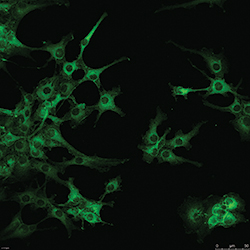
“The intervertebral disc experiences some of the largest magnitudes of stress and deformation of any structure in the body,” says Setton, who specializes in tissue regeneration and drug delivery. “It has no blood or nerve supply to help repair it, so it is the perfect system to study mechanics, because mechanics is the only thing that it sees or experiences.”
Setton’s lab has identified some novel ways in which cells interact with their environment, particularly in fibrosis — the thickening and scarring of connective tissue, usually as a result of injury — which can take place in organs, tendons, ligaments and the intervertebral discs.
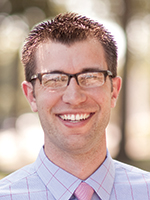
Spencer Lake
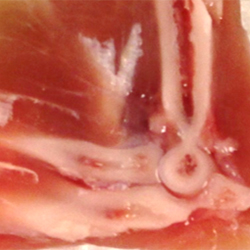
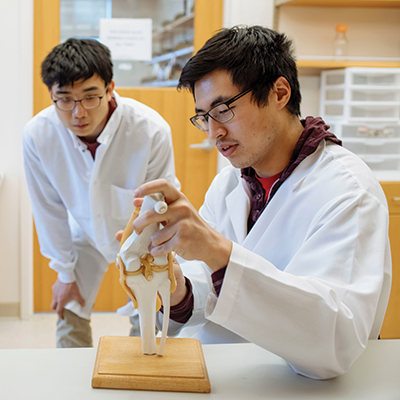
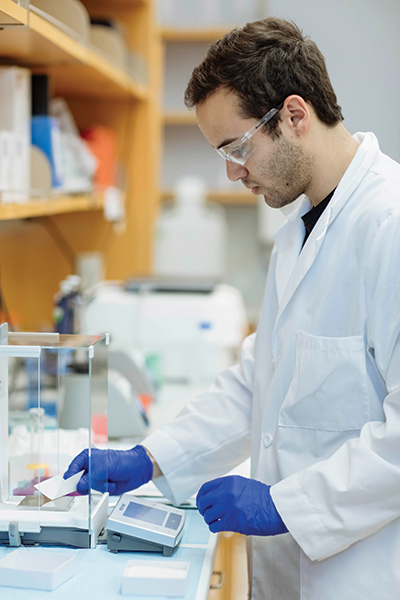
Lake, assistant professor of mechanical engineering and of biomedical engineering in Engineering and of orthopaedic surgery in the School of Medicine, studies how a displacement, load or strain at the large scale, such as a joint, muscle or tendon, gets transferred down to the smaller scales, such as the nanoscale. In addition, he studies how joints contract and shorten after a traumatic injury, particularly in the elbow, one of the most anatomically and biomechanically complex joints in the body.
“Following an injury, there is a fibrotic response that is driven by the cells in the tissues in the elbow joint,” Lake said.
“We’re trying to understand what tissues are involved in the response, what cells are causing that response and how we can intervene to limit that so that people maintain range of motion of their joints and are not debilitated by it,” Lake said.
The future
While researchers are creating models of injury and disease now with data they are collecting, physicians may soon use that data in addition to biological testing.
“Mechanical factors are going to play a bigger role in diagnosis,” says Philip Bayly, the Lilyan & E. Lisle Hughes Professor of Mechanical Engineering and chair of the Department of Mechanical Engineering & Materials Science. “Physicians will try to use the changes or differences in mechanical properties of tissue and diagnostics to determine, for example, if a stiff tumor is worse than a soft tumor. Or, based on the stiffness, are the tumors changing?”
Not only will it help with diagnosis, but treatments and therapies as well.
“Mechanobiology is going to lead to all sorts of new therapies for a variety of diseases, ranging from osteoporosis, osteoarthritis, atherosclerosis, cancer and 30 or 40 other diseases that have some type of mechanical interaction that either influences the disease or the condition or is a primary risk factor or cause for it,” Guilak said.
Currently, drugs are being developed that are likely ignoring some of the mechanical inputs that the body provides, Pathak said.
“If we have more information, then the drug development or screening or tissue engineering platforms will be better informed if you’re accounting for mechanical changes in the body,” he said. “That’s a very substantial outcome of this.”
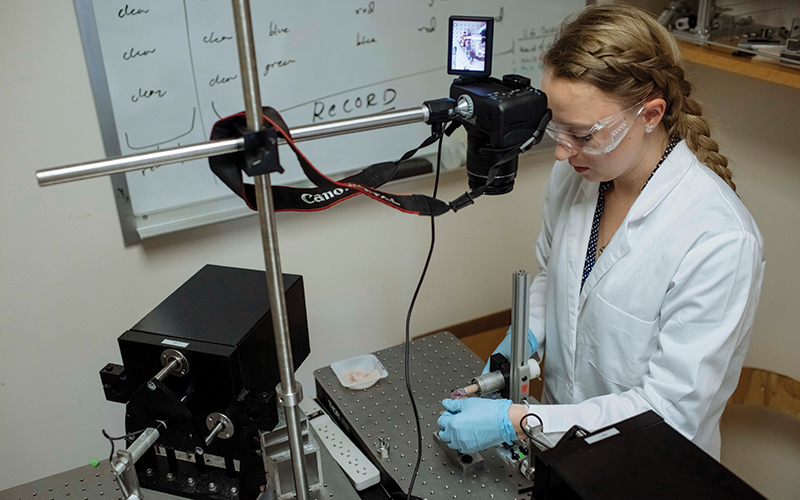
Science & Technology Center for Engineering MechanoBiology
Washington University’s role as a leader in the mechanobiology was confirmed in late 2016 when it was co-recipient of a five-year, $23.6 million grant from the National Science Foundation (NSF) to establish the nation’s first NSF Science & Technology Center on mechanobiology and one of only 12 STCs in the country.
The NSF Science & Technology Center for Engineering MechanoBiology (CEMB) at WashU brings together a consortium of leading mechanobiology researchers from WashU and University of Pennsylvania, as well as satellite sites at the University of Texas at Austin, the New Jersey Institute of Technology, Alabama State University, Bryn Mawr College and Boston University. These researchers are charged with developing mechanobiology as a discipline and discovering how mechanical force affects plants and human health in areas such as growth, development, stem-cell differentiation and cancer metastasis.
Guy Genin, professor of mechanical engineering & materials science and principal investigator of the CEMB at WashU, said research conducted by the center’s faculty has nearly limitless potential, ranging from stopping cancer cells from metastasizing to developing hardier crops and more sustainable growing methods that could boost food production.
“As this center unravels how plant and animal cells are controlled by force and by their mechanical microenvironment, we hope to apply this knowledge to develop technologies for new therapies, materials and agricultural technologies.”
— Guy Genin
“The mission of our center is to define how molecules, cells and tissues integrate mechanics within plant and animal biology,” Genin said.
Genin’s group seeks to understand and harness the mechanical control of cells. With Elliot Elson, the Alumni Endowed Professor in Biochemistry & Molecular Biophysics at the School of Medicine and a supporting faculty member in biomedical engineering and an affiliate faculty member in mechanical engineering; Tony Pryse, senior scientist in Elson’s lab; and Delaram Shakiba, a CEMB postdoctoral fellow, Genin seeks to uncover how a class of diseases called fibrosis can be controlled and reversed.
“Deciphering mechanobiological signals and responses is critical to curing fibrosis,” Genin said. “CEMB’s resources for this are remarkable.”
Genin also studies how mechanical signals of plant cells interact with Barbara Pickard, professor emerita of biology and a CEMB faculty fellow. They investigate how plant cells transduce sound and interact with their electromechanical environments with the aim of developing mechanical means of fighting insect invasion and drought.
Using mechanobiological technologies to optimize the form and composition of the plant cell wall, the team seeks to improve the efficiency with which biomass can be used for other products, such as biofuels or materials, which is the expertise of Marcus Foston, assistant professor of energy, environmental & chemical engineering.
“Plants have evolved mechanical feedback schemes that we can harness to improve production of biofuels and chemicals from them,” Foston said. “One example is tension wood. A plant that is pushed down tries to straighten out by adjusting its cell wall composition, including the amount of carbohydrates, which stiffen and straighten it.
“Even though tension wood has only 30 percent more carbohydrates, we were able to exploit the other chemical changes occurring upon tension wood formation to extract about three times the amount of sugar,” he said. “This means that tension wood formation increases the amount of carbohydrates for sugar extraction and biofuel production, but also makes those carbohydrates much easier to extract.”
Foston said the CEMB will allow researchers at all of the partner institutions to share the tools developed for animal systems and apply them to plant systems.
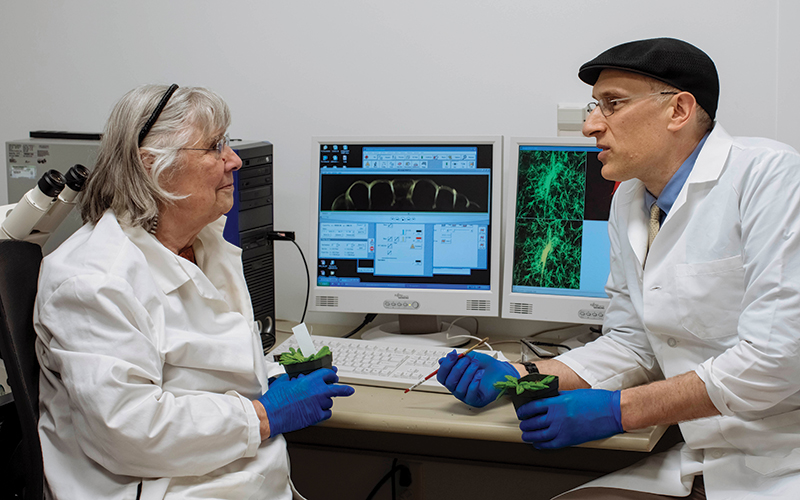